Last week we looked at many of the different energy creating technologies, and along the way learned about important concepts that drive energy decisions at small and global scales.
This week we will do deep dives into different energy storage and transportation technologies. These can be even more important than the energy generation technologies, because they can completely make or break whether those energy sources are economical and feasible.
Energy Storage
You can pretty easily find a way to store 3 gallons of gas in your garage or shed. But what if you need to store 3 BILLION gallons? This is the problem facing the people that are trying to modernize our power grid.
Wind and solar energy especially are wonderful sources of energy. No fuel is needed, initial capital costs have gone down by 5-20x in the last decade and are now lower than for many fossil fuel options, and O&M (Operations and Maintenance) costs per MW are becoming quite competitive. But the variability of these sources is a real grid-killer.
People don’t just want power while the wind is blowing or while the sun is shining. In Florida, every kW of installed solar panel capacity only produces 4kWh of energy each day, and….
…and boy, are energy units confusing. Before we can have any type of real discussion with hard numbers, we have to understand how energy industry people talk about electrical energy. It’s actually not all that confusing, and it’s kind of fun to think about. The first thing we need to do is differentiate between energy and power.
If you already understand MW and kWh, you can skip this section. If not, welcome to
Units of Energy Used by Utilities
First, let me tell you what they SHOULD have done, historically. In science, the standard unit of energy is a Joule. Energy is the ability to do work, the amount of stored “oomph” that it takes to do something.
If you connected a AA battery to an efficient electric motor and a good set of reducing gears, you could use one AA battery to lift a 100kg block of concrete about 10 meters1 into the air. That battery has enough energy to perform that much lifting (ignoring losses). Alternatively, you can use the energy in one AA battery to heat a 2 liter of Pepsi by 1 degree Centigrade.
The energy of a AA battery is just over 10,000 Joules. Joules is the correct unit of energy.
Power is how much energy you use per unit of time. So if I discharged an entire AA battery into a bottle of Pepsi in 10 seconds, the power of that situation is calculated as simply 10,000 Joules used in 10 seconds, or 1,000 Watts (a kilo-watt = kW). Of course, if you discharged a AA battery completely at the power rate of 1kW, the battery would melt or explode.
To recap, energy is the total “oomph,” and is measured in Joules. Power is how fast your are using that energy, the “oomph” per second, and is measured in Watts.
So if I wanted to measure how much energy your house uses in a month in order to calculate your electric bill, then I should measure that as Joules, or maybe kilo-Joules, or Mega-Joules, right? That’s how they SHOULD do it. But they don’t.
The power industry in America measures your total energy consumption in kilo-watt-hours (kWh). That’s right, it measures the power, which is energy per time, and then multiplies by time. Energy / time x time = energy. I find this frustrating, but that’s how it’s done.
If your home uses 72kWh, then that is 72kW times an hour. An hour is 3,600 seconds, so 72kWh is 72,000 x 3,600 = 259,200,000 Joules, or 259.2 MegaJoules (MJ). Now maybe you are like, “Hey, I used electricity all day, not just for an hour!” But this is just a way to sum up the total energy. If you mined bitcoin using 3 kW of power constantly for 24 hours, that would add up to 24x3 = 72kWh.
Or if you used your AC and TV for 12 hours at 6kW of power, that would be 12x6 = 72kWh.
1kWh typically costs around 9-15 cents, and that “kWh” on your power bill is exactly what we just described. It’s a measure of energy because it’s power times time, a kilo-watt times an hour. If your rate is 11 cents per kWh and you used 2,000kWh last month, then your bill will be something like $220 plus some taxes and fees.
In large grid-scale batteries, they discuss two things: the size of the batteries in MegaWatts (MW), and the size of the batteries in MegaWatt-hours (MWh). Journalists get this mixed up all the time, because it’s confusing.
But now you can probably guess how to figure all this out. Batteries store energy. So the total amount a big battery bank may store is measured in MWh. Let’s say a huge battery installation holds 50MHh of energy, which means it could output a MegaWatt for 50 hours before being completely drained.
But then the battery will also have a power rating. There is a maximum that it can discharge out onto the grid at once without overheating. This might be 5MW, or 5 million Joules of energy per second. So this mean that this 50MWh battery can output a max of 5MW constantly. If it starts full, then this means it can output 5MW for up to 10 hours before it’s fully drained.
If you have a 9kW solar panel system, that means it will produce a maximum of 9kW of power (9,000 Joules per second) when the sun’s position is just right on a sunny day.
You are now in the top 5% of the population in understanding units of energy the way power utilities use them.2
*** End of Units of Energy Section ***
Ok, now that everyone is on the same page with electrical energy/power units, we can discuss the storage options.
One thing a lot of people are surprised by is the scale of the energy delivered by the electrical power grid. In 2020, Americans use about 100,000,000,000,000,000,000 Joules of electrical energy. That is 100 quintillion Joules. This comes out to about 4,000 TeraWatt-hours (TWh) for the year, or about 12 TWh every day, just in the US.
If we want to move to 100% solar or wind power, then we need to have at least enough storage to cover nighttime plus the worst possible cases for high daytime cloud cover and low wind strength. And then on top of that, you have to factor in the worst case scenario for a string of days which may each have a deficit of power for perhaps two weeks. And then there are seasonal variation. Much of the contiguous US has only 10 hours of sunlight each day during the winter, and large sections of Alaska have zero hours of sunlight for months.
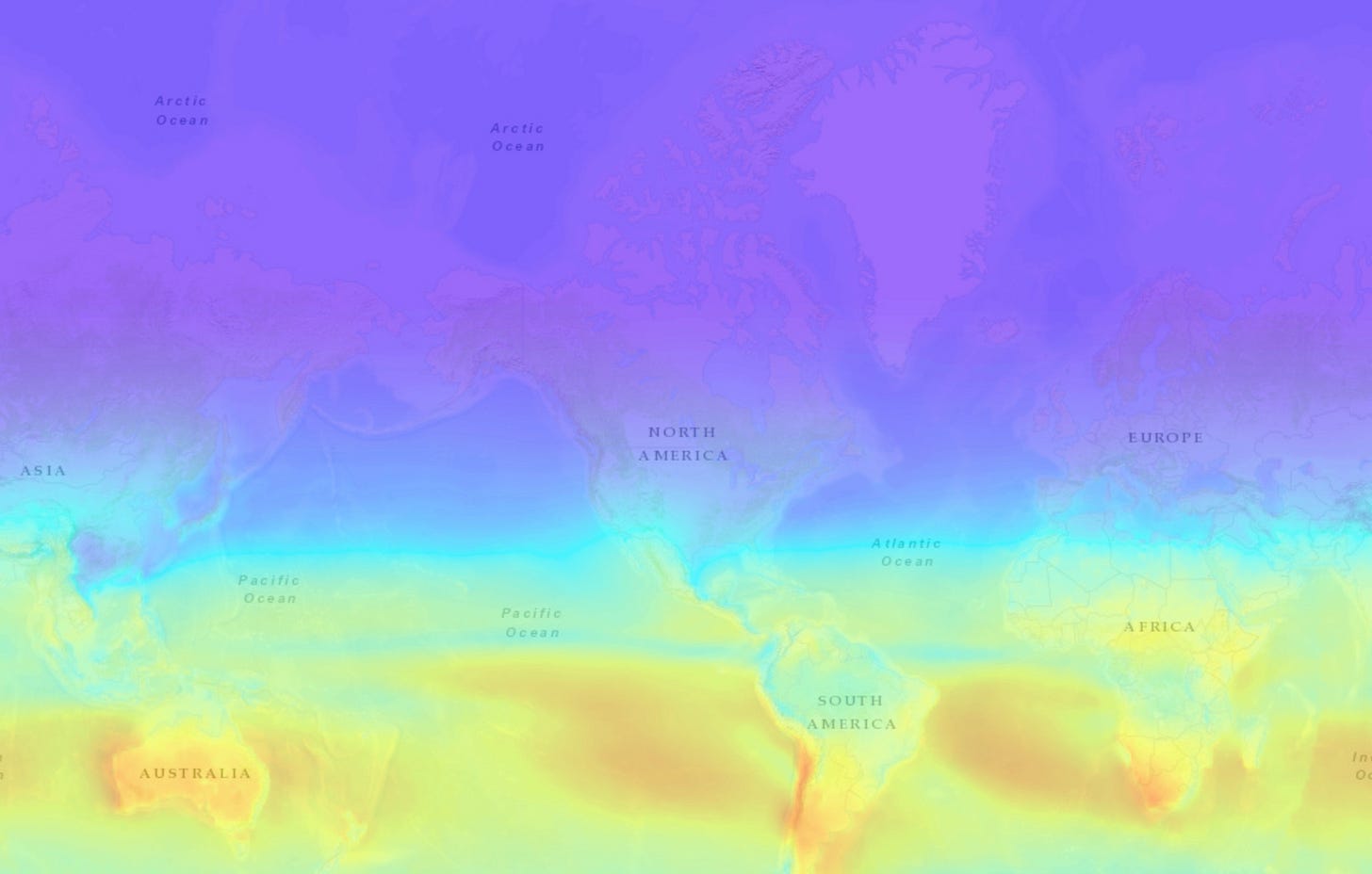
On top of this, the entire US grid is not connected together. A large part of Texas and some of Oklahoma make their own completely separate power grid, and even the connected parts of the grid have power bottlenecks. There could be a really great, sunny month in Arizona, but there might not be enough transmission capacity to send all that extra power to Colorado.
So this means that if we want to go completely onto solar and wind, we need at least 36 hours of energy storage to take account of the worst case scenarios, we need to have 18 TWh of energy storage. That is 18 million Megawatt-hours of energy storage. Let’s call it the Eagle Screech battery, because it would be an awesome American achievement that enables America to go to 100% renewable energy.
To give you an idea of how large this amount is, the total battery production capacity of the entire planet is about 0.7 TWh per year.
And this includes making batteries for laptops, cars, toys, and grid storage applications.
Now that you understand the scale of the problem and the units used to measure such things, we can evaluate different storage and transmission technologies.
Lithium Ion Battery Storage
The good news is that batteries are amazing storage devices. They are fast, fairly reliable, and can be arrayed into many different storage sizes. You can have a 0.02kWh battery for your phone, or you can have a 2,000,000kWh battery array for your energy grid. That flexibility is not a trivial thing, and the cost is not that different even if the size varies by 6 or 7 orders of magnitude.
One of the very best things about lithium ion batteries is the round trip efficiency. This is probably the most important factor for any storage method, and lithium batteries are the best at this. The round trip efficiency is how much you energy you lose as you charge and then discharge the storage device. For batteries, this round trip efficiency is very close to 95% or even higher. You don’t lose hardly any energy while putting it in or taking it out. Alternatively, if you wanted to store energy by boiling water to make high pressure steam and store it in a container, and then use a steam turbine hooked to a generator in order to get that energy back the next day, your round-trip efficiency will be around 25%, and this will change to be even worse the smaller the system is.
There is one little twist when combining batteries and grids. Batteries are DC (direct current) storage devices, meaning you use a static voltage (and thus current) to charge them, say 4.2 volts.3 But our electric grids all use AC (alternating) current. AC means the voltage and current go up and down (or back and forth) in the wire constantly, wiggling like a sine wave.
So when you discharge a battery onto the grid, you first need to go through something called an inverter, which turns the DC battery voltage into AC grid voltage at the correct frequency, 60Hz. Inverters have gotten quite efficient in the last decade or so, regularly reaching 95%. But this means that if you have 100kWh stored in your battery, it can only put around 91kWh onto the grid (or into your home) as useable AC power.
And it works the other way, too. If you want to use grid power to charge your battery, the converters going the other way (from grid AC to battery-charging DC) are also about 95% efficient. So if you want to use 100kWh of grid energy to charge a battery, then only 95kWh makes it into the battery, and then when you put that energy from the battery back onto the grid, only a little more than 86kWh actually makes it back onto the grid. So for grid storage, the round trip efficiency of batteries is about 86%.
But solar panels output DC, so batteries absorb that without having to go through inverters. Wind turbines produce AC. So they can put energy directly onto the grid with no inverters. But you need those AC-to-DC converters in order to put wind power into a battery.
This is why solar + battery is such a powerful option. The solar panels output voltage in a way batteries love, so the trip from solar panel to battery to grid is around 90-94% efficient. You are making really good use of your solar panels and you have scalable, fast, and long-lasting storage.
But of course there are negatives, like cost. Currently, batteries cost around $400 per kWh of storage. For fun, this means that the 18TWh Eagle Screech battery will cost $7.2 Trillion. Which is a major purchase, but probably pretty reasonable given the awesome electric sourcing flexibility it would allow. The real problem is that all the world’s battery factories would have to be dedicated to that one order only for 25 years in order to fulfill it. So battery production is a major chokepoint for really widescale battery deployment. It can’t happen in just one decade.
But can we mine enough lithium, nickel, and cadmium to support that? Yes, we can. It’s a popular misconception that China controls the world’s lithium reserves. They simply have the cheapest supply (due to low labor costs and lax environmental regulations). But lithium was the third-most abundant element created during the Big Bang, and is everywhere. The US used to produce plenty of lithium until China flooded the market with cheaper lithium. The world reserves of lithium are estimated at 65 million tons currently, and that supply can go up based on demand. Lithium is even fairly abundant in seawater. 1kg of lithium will produce about 6kWh of battery storage, so to make our 18TWh Eagle Screech battery, we would need about 3 million tons of lithium, or about 5% of currently known reserves.
For every kilogram of lithium in a battery, you need bout 4kg of nickel and 2kg of cobalt, though both those proportions vary widely and will vary widely in the future. There is a lot of research in this area to reduce the need for these elements, but even assuming all that research fails, our US battery of awesomeness will still only need 15% of world nickel reserves. We do need to move away from cobalt because this battery project would consume almost all the world’s cobalt reserves. Thankfully, Samsung and Tesla have already found ways to completely eliminate cobalt going forward.
So really, there isn’t a huge deficit of necessary elements for DRASTICALLY increasing battery production. And lithium is mostly mined by evaporating water out of brine created from dirt washed down from desert cliffs, so the environmental impact is not terrible (though not negligible, either).
Prediction: Lithium ion batteries are the future of energy storage. They are fast, scalable, not that expensive (and getting cheaper). Nothing else can really compete with them, given what we know right now.
For completeness, we will look briefly at other energy storage devices before we look at power transmission over long distances.
Flow Batteries
These batteries are interesting because they are mostly comprised of liquids that can be “charged up” and stored in tanks. A reaction membrane of almost arbitrary size can be used to get as much power into or out of the flow batteries as one desires. When you bring the two fluids into contact with either side of the membrane, ions travel across the membrane and produce electrical power. You can reverse this process by applying power to the membrane to re-separate the ions to “recharge” the fluids.
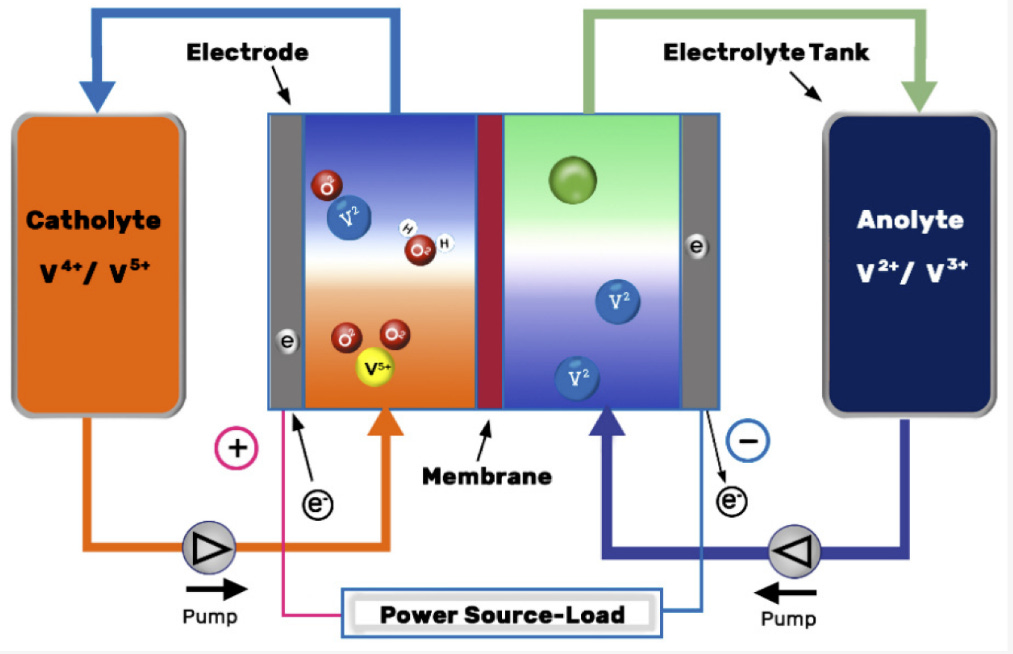
The problem is that the round trip efficiency of flow batteries isn’t great, being around 70%. This is much worse than the 95% for lithium ion batteries. Cost is also a problem, with vanadium flow batteries currently about 1.5-3 times more expensive than lithium ion batteries. There are some speculative flow battery technologies that replace vanadium with iron, and those could become as cheap as $25 per kWh. This would be incredibly dramatic, but I read this as more investor-bait than reality. We’ll see. One should keep an eye on flow batteries. Because even if the round trip efficiency is moderate instead of great, the cost may become very low, and that makes up for the round trip efficiency problems.
Right now, the largest vanadium flow battery being constructed is 8,000kWh at a cost of about $1,700 per kWh, which is four times the expense of a similar lithium ion battery installation.
Prediction: Flow batteries might take some real market share of the grid storage market from lithium ion batteries, if they ever become 30% or more cheaper than lithium ion batteries. There are some indications this might happen, so they should be watched carefully. Look for large scale implementations (greater than 20MWh). If those occur, then this technology might be ready for rapid expansion.
Pumped Hydro
If you have a great place to store large amounts of water up high, then you can store a lot of energy very efficiently by using electricity to pump water uphill, and then run it through a turbine (like in a dam) to get that electric power back. Pumped hydro is incredibly inexpensive, and it has a good round-trip efficiency of around 80%. And you might be surprised to know that pumped hydro is currently the number one energy storage technology in America, comprising almost 22GWh of storage in 2021. This means pumped hydro accounts for a whopping 92% of current energy storage in the United States.
But you can’t very easily expand it. Most pumped hydro “facilities” are accidents of geography. This is because the energy density of pumped hydro is incredibly bad. By “energy density,” I mean the amount of energy stored per unit volume. Lithium ion batteries can store a lot of energy in a very small volume, around 0.5kWh per liter. Remember how I said a AA battery can lift a 100kg weight 10 meters? Well, that means that the potential energy density created by lifting water up high is actually very low. If you raise one liter of water up 100 meters, then you only are storing about 0.0003kWh of energy. That means lithium ion batteries take up almost 2,000 times less space than a 100 meter tall pumped hydro solution.
In order to make our Eagle Screech battery, we would need to pump almost 100 trillion gallons of water 100 meters high. This could be done by pumping all of Lake Eerie up into the air as high as the Statue of Liberty’s torch. This would probably reduce local property values. And remember, this only stores enough pumped hydro to run America for 36 hours.
Prediction: Due to low energy density and lack of favorable new geographic areas for implementation, pumped hydro will not be a growing segment of energy storage and will soon be surpassed forever by other technologies.
Pumped Air
Some people want to fill giant caves with lots of high pressure air, storing electricity as pressurized air (using electric air pumps to fill the cave). Then you can release that pressure and make the air go through a turbine, recovering the electricity.
No. Too big of spaces, too dangerous, too few feasible sites.
Prediction: No.
Heating Stuff
People have looked into using electric heaters to heat rocks, sodium, salt, and other items. Then when you want the energy back you blow water or air over that hot stuff and run a steam or air turbine to turn that heat into electricity.
These have round trip energy efficiencies of well below 60%, usually below 45%. In most cases, the temperature differential is low and/or variable, meaning efficiency can is quite variable and can thus fall even lower.
Prediction: No.
Flywheels
If you spin a big cylinder super fast, to almost the breaking point of the material it’s made of, you can store a LOT of electricity in a small space, stored in the angular inertia of the spinning cylinder. And you can directly attach the flywheel to an electric motor to spin it up, and an electric generator to spin it down.
Using a big bank of these, you could store a significant amount of energy, and the round trip efficiency is pretty good, on the order of 90%. One of the really great things about flywheels is that they can dump a lot of energy all at once, but basically going from spinning very fast to not at all in just a few minutes, while connected to an appropriate generator configuration.
Unfortunately. the outside drags air with it via friction. But you can just encase the flywheel in a vacuum, and that solves many problems. There is also the concern of constant bearing friction. Fortunately, there are companies working on superconducting bearings for flywheels, which helps the flywheel stay spinning longer, losing less of its energy to the heat of friction.
The biggest commercial flywheels are currently on the order of 100kWh, and they spin extremely fast, around 45,000rpm. This is a fairly dangerous way to store very large amounts of power, because there is a lot of kinetic energy stored in a big moving item. That said, the same is true of natural gas turbines. Still, if we wanted to make even a modest 50MWh energy storage facility, we would need 500 flywheels. Eagle Screech would require hundreds of millions of flywheels. Flywheels, even in a vacuum with the best bearings, will still lose about 5% of their energy daily, or about 1/3 per week. That’s not horrible.
But a big problem is the spin rate. Electric grids want their power at 60Hz or 50Hz, and flywheels must spin MUCH faster than this in order to be useful for energy storage. So complex, continuously variable gearboxes must be used between the flywheel and the generator, and this adds a lot of cost and a lot of friction for extra losses.
Due to this, it appears people simply don’t have the stomach for massive amounts of flywheels because of the safety factor. Some power-time-shifting facilities are profitable, thought. Smaller flywheels might very well be useful in electric car chargers in the near future, because they can store a lot of energy and dump it in just a few minutes into a car, charging it faster than almost any other method. Those electric car chargers don’t care about the frequency because they “rectify” the highly variable AC power into DC power for the car’s batteries anyway. Hmmmm…..
Prediction: Flywheels aren’t widely adopted for AC power storage and generation due to issues related to gearboxes and the danger of a very fast spinning cylinder. But they do have some very interesting niche applications, and if you need DC power stored and recovered, they might be useful if you have very efficient AC to DC and DC to AC converters.
Green and Blue Hydrogen and Natural Gas
One can use excess electrical and heat energy to turn natural gas into hydrogen for energy storage (blue hydrogen). Once can also turn water into hydrogen for energy storage using direct electrolysis, which basically splits the oxygen from the hydrogen electrically (green hydrogen).
The round-trip efficiency for all of these is abysmal, typically on the order of 35% or worse. These are awful ideas that are only amplified by how difficult it is to store and use hydrogen. It takes up more space than any other gas. Sure, you can cool it to be a liquid, but that cooling takes a tremendous amount of energy because you have to cool it to just a few dozen degrees above absolute zero (at ambient pressures). And hydrogen will leak out of almost everything because it’s pretty much the smallest molecule except gaseous helium.
A lot of people talk about a hydrogen economy, but for the reasons above and many more I don’t have space for, hydrogen is an awful idea for energy storage and transportation.
Prediction: There will never be a hydrogen economy on earth’s surface. The best possible round trip efficiency is still worse than 50%, due to fundamental physics. No technology can recover from that, and hydrogen has a bunch of other logistical and materials problems as well. It may be useful in space, but I doubt that also.
Energy Transportation
I am not going to talk about transporting fossil fuels in pipelines or cargo ships here, as those fossil fuels are typically not energy that humans have actively stored, but rather dug up. Thus, fossil fuels don’t directly enable new energy sources in their storage or transportation, though as we discussed last week natural gas helps stabilize the grid as we add more solar and wind. Instead, we will talk about the very fast transportation mechanisms of transporting energy electromagnetically and electrically.
Beamed Power
This is often mentioned as an enabling technology for space-based solar power (SSSP). Solar panels in space can “catch” about 40% more energy per square meter than on the ground at high noon, and are never blocked by clouds. At high enough orbits, they are rarely blocked by the Earth, either.
But we can’t run a wire from these satellites to ground, so we must use beamed power of some sort to get the energy to the ground. Infrared gets blocked by clouds and water in the air. Optical (visible light) beaming will blind people in the beams, or even those that look at anything that accidentally scatters the beams. Therefore, the much longer wavelengths of microwaves are postulated for transmitting power wirelessly over distance.
As we discovered in an article about using orbital lasers to mine the moon, any orbital transmitter that wants to make a tight beam must have a transmitter that is around 1,000-10,000 times wider in diameter than the wavelength being used. The best and safest microwave wavelengths are around an inch in size. So the transmitter might need to be 5,000 inches wide, which is 400 feet in diameter. This means that the active electronic area of a phased-array antenna must be incredibly large, and this means more weight that must go to space, more things to break, and more intricate engineering to perform and get working as a space-based facility. And one that is very difficult and expensive to fix if anything breaks.
Further, we don’t want tight beams of intense energy aimed at the earth because of reflection dangers and mis-aiming dangers. So you spread it out a bit anyway, but this means the ground receiving stations must be extremely large. In order to receive 50MW of power at reasonable levels, you need a facility on the order of 10 square kilometers. This is much larger than a 50MW solar field, and even a solar field operating at 25% total day-round efficiency (meaning, you average the power over all the nighttime and clouds) will be many times smaller to produce 50MW x 24 hours = 1200MWh of energy per day than a 50MW SSSP receiver station.
Some estimates show that SSSP can be economically competitive once launch costs get to below $200/kg (which might happen with SpaceX’s Starship), but I think that the cost of ground stations and the large size and inability to maintain space-based infrastructure would require a launch cost much, much lower to make this economically.
What about using it as ground-to-ground transport? If we stay in-atmosphere for hundreds of kilometers, curving around the earth with microwave repeater stations, then the losses (and dangers) of that microwave beam will be much worse than regular electrical cables will be.
Prediction: Beamed power, whether space-to-ground or ground-to-ground is extremely costly, potentially catastrophically dangerous (death beams from space) and very likely uneconomical or technically infeasible.
Land-Based Power Lines
These work really well! They act as concentrating tubes for electrical field transmission, not just as electron pipes. For long distance transmission of electrical energy, we’ve learned that the higher the voltage we use, the fewer losses there are per kilometer of length. This is because to send the same amount of power (let’s say 100MW), you can either send low voltage/high current (say, 1,000Volts and 100,000 Amps), or high voltage/low current (Say, 100,000 Volts and 1,000 Amps).
But the power losses go up with the square of the current. So the high current version in this example would have literally 100x100 = 10,000 times the losses than the low current version. This is why long electrical transmission lines are boosted to really high voltages. The losses go way down.
So humans have learned to make higher and higher voltage lines for long distance in order to vastly reduce the losses in the lines. We can also cut the losses in the lines by half or more if we transmit using DC power instead of the normal AC power. Currently4, 99%+ of transmission lines are AC, but DC high voltage is an up-and-coming technology.
High voltage transmission lines can have low enough losses to reasonably go up to 2,500 km, though it’s not optimal. DC lines have the promise to send power with acceptably low losses up to 6,000km.
And they are very “fat pipes” in terms of the power density. A single Chinese DC line (at a whopping 1.1 million volts) was shown to be able to transmit 12 billion watts continuously on a single line.
Which opens up several VERY interesting paths forward.
That’s the end of the survey of power storage and transmission technology. But let’s quickly review the important universal power grid concepts that we’ve learned this article:
Utilities use confusing power/energy units, but now you understand them.
We learned what “round trip efficiency” is and why it’s very important for energy storage.
Lithium-ion batteries work great and are environmentally feasible. They’re just pretty expensive (but getting cheaper).
We learned the incredible scale of grid storage it would take to move to 100% renewables.
Other types of energy storage are mostly infeasible at grid scale.
Flow batteries have some promise, but are unlikely to make a grid-scale difference unless they get 30% cheaper than lithium-ion batteries.
“The Hydrogen Economy” will very likely never happen due to poor round trip efficiency, storage, materials, and density.
The best way to move power is power lines, but DC high voltage adds some interesting new features.
I have spent two of the longest articles I have ever written just to make sure everyone understands how to think about energy grids, to know what matters.
Next week is the payoff, where we use all this knowledge to put forth a vision of the best possible energy grid and energy technologies for 50 years from now.
We will finally dive into the Deep Future of Energy.
Or, in different units, lift 1 hogshead of mead up 1 rod.
There’s also another unit called BTU (British Thermal Unit), which is 1,055 Joules. There are 3,412 BTUs per kWh. A quadrillion BTUs is called a quad. Sometimes people might say a country used 127 quads last year. People that hate rational units.
Volts are a measure of electrical potential energy. If you have a high voltage between two pieces of metal, this means that a LOT of current wants to flow between them. If you have low voltage, only a little bit of current wants to flow between them. Current is how many electrons (sort of) want to pass by a certain point per second. The power (watts) is known simply by multiplying the voltage times the current. High voltage, High current circuits have a lot of power going through them. When you rub your hair on a balloon, there is fairly high voltage (around 1,000 volts), but basically zero current, so not much power flows. But then if you touch a doorknob, the current will flow and hurt your finger for a tiny fraction of a second.
Another home-run Joshua!!
I remember touring the 1,100 MW pumped-storage hydro-electric power plant, Blenheim-Gilboa, in upstate New York back in '86 and was very impressed by it. The plant paid for itself in 7-years, simply by the difference in night vs daytime power prices.
Keep up the great work!
Terry Terezakis